Gas sensors have played a crucial role in numerous industrial processes over the past few decades. These sensors are designed to detect and monitor the presence of gases in the air, finding applications in safety systems, environmental monitoring, and process control. The development of gas sensors has seen significant advancements over time, resulting in sensors that are more sensitive, accurate, and reliable than ever before. This article explores the key developments in sensor technology for gas detection, examining different types of sensors, the challenges faced in their development, and the new technologies currently being developed to meet the needs of modern industries.
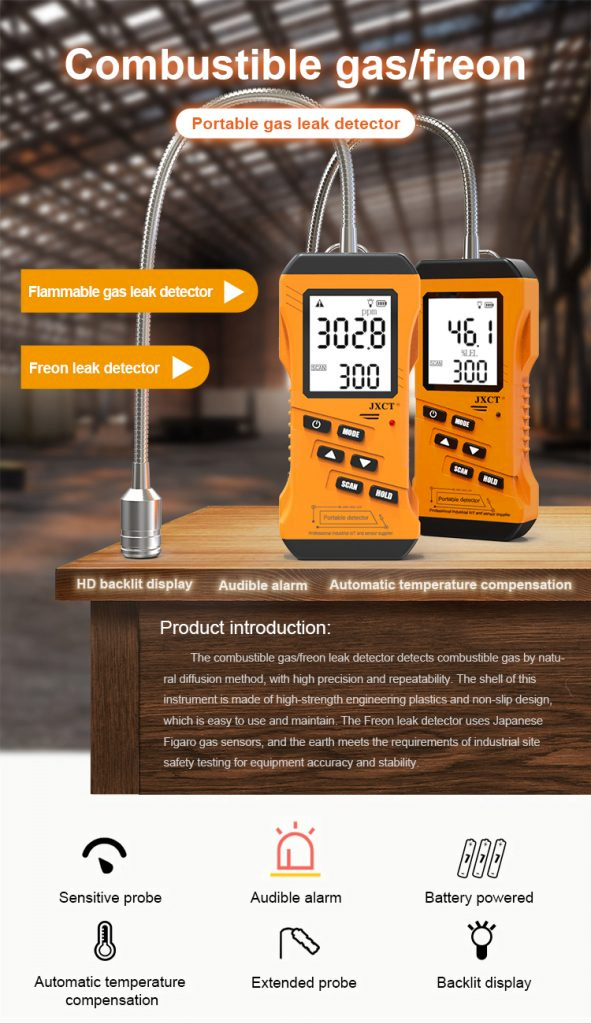
Types of Gas Sensors
Several types of gas sensors are commonly used in industrial applications. These include electrochemical sensors, catalytic sensors, infrared sensors, and ultraviolet sensors. Each type of sensor is designed to detect different types of gases and has its advantages and disadvantages.
Electrochemical Sensors
Electrochemical sensors operate based on the principle of electrochemistry, where a chemical reaction occurs between gas molecules and an electrode. This reaction produces an electrical signal that is proportional to the gas concentration. These sensors are widely used to detect toxic gases such as carbon monoxide, hydrogen sulfide, and ammonia.
The primary advantage of electrochemical sensors is their high sensitivity and selectivity to specific gases. However, they can be susceptible to temperature drift and cross-sensitivity issues, where they may respond to gases other than the target gas. Researchers have been working on addressing these challenges through advancements in sensor design and multi-sensor data fusion techniques. For instance, a study in the Instrument Technique and Sensor journal detailed a multi-sensor data fusion algorithm using a linear neural network to improve the detection of SF6 gas decomposition products, specifically addressing cross-sensitivity issues in electrochemical sensors.
Catalytic Sensors
Catalytic sensors work by detecting gas molecules that react with a catalyst material. When the gas molecule reacts with the catalyst, it produces heat or a change in electrical properties. These sensors are commonly used to detect flammable gases such as methane, propane, and hydrogen.
The main advantage of catalytic sensors is their ability to detect a wide range of flammable gases with high sensitivity. However, they can be less selective and may respond to non-target gases, leading to false positives. Researchers are continuously working on improving the selectivity of catalytic sensors by optimizing the catalyst material and sensor design.
Infrared Sensors
Infrared sensors detect gas molecules based on the absorption of infrared radiation by the gas molecules. Each gas molecule absorbs a specific wavelength of infrared radiation, allowing the sensor to identify the gas molecules present in the air. These sensors are commonly used to detect carbon dioxide, carbon monoxide, and hydrocarbons.
Infrared sensors offer high selectivity and can operate at room temperature, making them suitable for various applications. However, they can be more expensive and require more complex calibration procedures compared to other sensor types. Advances in materials science and sensor design are focusing on reducing costs and improving the performance of infrared sensors.
Ultraviolet Sensors
Ultraviolet sensors work by detecting gas molecules that absorb ultraviolet radiation. When the gas molecule absorbs the radiation, it produces an electrical signal that can be measured by the sensor. These sensors are commonly used to detect ozone, nitrogen oxides, and other gases that absorb ultraviolet radiation.
Ultraviolet sensors are highly sensitive and can detect gases at very low concentrations. However, they are limited by their ability to detect only gases that absorb ultraviolet radiation. Researchers are exploring new materials and designs to broaden the detection range of ultraviolet sensors and improve their overall performance.
Challenges in Developing Gas Sensors
Developing gas sensors that can detect a wide range of gases with high sensitivity and accuracy is a challenging task. One of the main challenges is designing sensors that are selective to the target gas and do not respond to other gases present in the air. This requires careful selection of sensing materials and optimization of the sensor design.
Another challenge is the need for sensors to operate reliably in harsh environments, such as high temperatures, humidity, and pressure variations. Researchers are continuously working on developing robust sensor designs and materials that can withstand these conditions while maintaining high performance.
Cost is also a significant factor in the development and deployment of gas sensors. Reducing production costs while maintaining high sensor performance is a key objective for researchers and manufacturers. Advances in materials science, manufacturing techniques, and sensor design are focused on achieving this goal.
Recent Advancements in Gas Sensor Technology
In recent years, there have been several significant advancements in gas sensor technology that have improved detection capabilities.
Graphene-Based Gas Sensors
Graphene-based materials have shown great potential for developing gas sensors due to their unique band structure, large specific surface area, and high carrier mobility. Researchers have developed chemoresistor-type NO2 sensors based on chemically modified graphene materials (CMG), including sulfonated reduced graphene oxide (S-G) or ethylenediamine-modified reduced graphene oxide (EDA-G).
These sensors exhibit a stronger response to NO2 gas than reduced graphene oxide (rGO) counterparts, with responses ranging from 4.3 to 16.4 times higher. The CMG-based sensors can spontaneously recover to their initial states by flowing N2 gas, without the need for UV/IR light illumination or thermal assistance. The easy fabrication of these sensors makes them attractive for ultrasensitive detection of NO2 gas. This work indicates that chemical modification is an effective approach for improving the performance of rGO in gas-sensing applications.
Wearable Gas Sensors
In the era of the Internet of Things (IoT), wearable technologies are becoming an indispensable part of daily life. Highly sensitive, flexible, and stretchable electronic gas sensors are gaining significant interest due to their applicability in wearable electronics applications. Wearable gas sensors are designed for real-time, highly sensitive, and selective detection of hazardous gases at room temperature. They can be laminated onto the human body or integrated with body-worn textile or other consumer products.
Wearable gas sensors utilize carbon nanomaterials, conductive polymers, 2D nanostructured materials, and their composites. The critical challenges for the future development of wearable gas sensors include improving sensor selectivity, reducing power consumption, and ensuring long-term stability. Wearable gas sensors have great potential in environmental monitoring, healthcare, public safety, and food quality monitoring.
Semiconductor Gas Sensors
Semiconductor gas sensors offer high sensitivity and a broad detection range. Recent research has focused on utilizing low-dimensional semiconductor materials and their composite structures to reduce sensor working temperatures. The discovery of new semiconductor room-temperature gas-sensing effects and enhancement mechanisms has the potential to break through the technical bottlenecks of low power consumption and integration in semiconductor gas sensors.
One study investigated the use of PbS quantum dots/MoS2 nanosheet low-dimensional semiconductor composite structures to enhance the room-temperature gas-sensing effect. The composite structure effectively combines the rich active sites of quantum dots and the relatively high mobility of MoS2, resulting in significant room-temperature gas-sensing effects. The sensor exhibited high sensitivity, fast response and recovery times, and good selectivity towards NO2 gas.
The preparation method and process conditions are mild, facilitating the full utilization of the large specific surface area of low-dimensional composite semiconductor structures in actual devices, thereby improving room-temperature gas-sensing performance. This research demonstrates the potential of sulfide semiconductors and their low-dimensional composite structures for developing new semiconductor gas sensors.
Conclusion
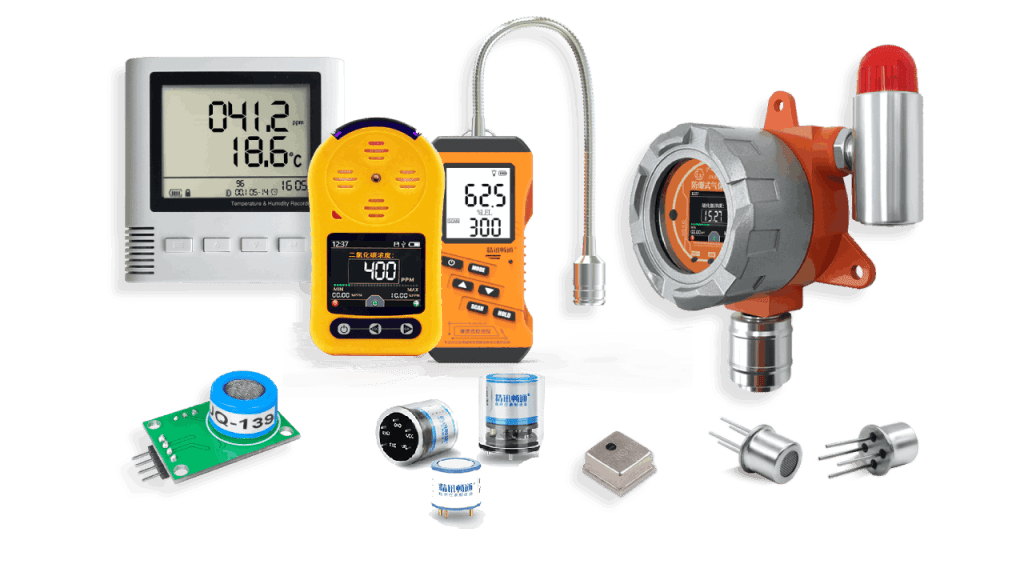
Advancements in gas sensor technology have significantly improved detection capabilities over the past few decades. Different types of sensors, including electrochemical, catalytic, infrared, and ultraviolet sensors, offer unique advantages and applications. However, challenges such as selectivity, robustness in harsh environments, and cost remain significant obstacles in sensor development.
Recent advancements, such as graphene-based sensors, wearable gas sensors, and low-dimensional semiconductor sensors, have shown great promise in overcoming these challenges. As research continues, we can expect further improvements in gas sensor technology, leading to more sensitive, accurate, and reliable detection of gases in various applications. The future of gas sensor technology holds exciting possibilities for enhancing safety, environmental monitoring, and industrial processes.